Due to the lower air density at higher altitudes your engine may run slightly rich and therefore cooler. The general rule of thumb is that you may advance your timing one degree for each thousand feet of altitude. And with that stated let me offer some personal advice. If you do not have a degree wheel, and are not running electronic ignition the chances are that your timing will wander a bit even under ideal circumstances, thanks to the plug gap, wear in the distributor shaft, rubbing block and so forth. If you intend to operate above 5,000 feet elevation for a prolonged period of time (i.e., a move to Denver, etc) then by all means, re-time your engine. Otherwise, leave it alone, unless it gives tells you in an obvious way that it would prefer a different setting, in which case crank in a degree or two . . . whatever the engine likes . . . to return its operation to harmony with your driving habits, load carried, type of gas and, for all I know, the color of your hair and eyes. The point is, the settings in the manuals refer to new engines operated under a set of fixed conditions. Reality is seldom so kind, yet the engine is always willing to let you know when it is happiest.
The symptoms of altitude sickness usually resemble retarded timing; lack of power, poor economy, possibly a bit of black smoke or start-up or when attempting to accelerate. However, if you are running a hot CDI or other all-electronic ignition module, the symptoms may be partially masked by the cleaner burn and longer spark duration provided by most CDI’s ( which are easily retro-fitted if you’re not presently using one. Coupled with platinum plugs, the combo virtually eliminates tune-ups for air-cooled veedubs.)
You may put aside any fears of high-altitude (i.e., low-pressure) vapor locking (as opposed to high temp vapor locks). Since about 1980 all automobile fuel sold in the United States has a vapor pressure that allows it to be used safely to about 12,000 at 100 degrees Fahrenheit (an unlikely combination).
For the past twelve years the Experimental Aircraft Association has been using mogas in aircraft, reporting the results to the FAA and the EAA members. Several certified aircraft engines are now permitted to use mogas, or ‘tractor’ gas as pilots tend to call it. Works fine, even in old Volkswagens; no problems with low-pressure vapor-locking.
(Ed. Note: This is NOT true if the gasoline contains alcohol. Not only does alcohol reduce the energy content of your fuel, it promotes vapor lock and carburetor icing. R.S.Hoover, 2006)
Thursday, November 23, 2006
VW - Chamber Volume
.
Holding chamber volume to .1cc is like running a four minute mile . . . not everyone can do it and even those who can often choose to take the bus.
Unless you’re going for the gold, the added time and expense of nailing down the chamber volume to a perfect, repeatable figure within .1cc across all four jugs is really gilding the lily. It has taken me as long as a week to match a set of heads to 0.1cc, whereas getting them within 1.0cc ( plus or minus 0.5cc) is pretty easy.
Normally, when making small adjustments to your chamber volume you do so by sinking one of the valves. If you’ve got a lot of volume to make up, you sink the intake, but never very much. You want the intake valve to keep its head up. On the other hand, the exhaust valve can be down in a well and still work, thanks to the pressure during the exhaust cycle. But adjusting valve depth is not a trivial task. You need a set of stones and the tools and the skills. And you must be accustomed to working to very close tolerances. Go a bit too far and you’ll end up having to do the other three combustion chambers over again, to match your mistake.
You’ll encounter the same difficulties when you try to keep your deck height to some small value. Deck height determination starts before the engine is assembled. You need to determine the precise center-to-center length of each rod . . . they vary according to how the big end is honed . . . and on each piston, the distance between the wrist-pin bore and the head of the piston. You also must measure each of your cylinders using a surface plate and surface gauge. You do your first pre-assembly by matching ‘short’ rods to ‘long’ pistons in an effort to make all of equal length but you don’t really know anything until the crankcase is torqued and the cylinders seated with a dummy head, a plate with spacers that allows you to torque the cylinder studs to spec. Deck height is measured through a hole in the plate.
The trick here is to arrive at a consistent height for all of the cylinders while you maintain the same deck height. It is virtually impossible to do this without having a lathe, since the final dimension is usually achieved by shaving a few thou off the heads of the ‘fat’ pistons, but you can shuffle components to arrive at the best average.
Deck height is the most critical of your volumetric balance dimensions because a small dimensional change results in a large change in volume.
If you’re building a plain vanilla engine, measure everything but do not attempt to balance chamber volume until you’ve done at least the first pre-assembly to determine deck-height. Often times you’ll be able to balance a big chamber with a small deck-height and arrive at a good average volume across all four jugs.
A key point to keep in mind is that your engine will run with some amazing imbalances in both mass and volume, but it does so at an equally amazing cost. An out of balance engine is destined to live a short, expensive life whereas one that is balanced with respect to both mass and volume uses less fuel to produce the same power and has a useful life considerably longer than it’s mass-produced cousins. And these are improvements that are built-in for the life of the engine, repaying their cost many times over.
Holding chamber volume to .1cc is like running a four minute mile . . . not everyone can do it and even those who can often choose to take the bus.
Unless you’re going for the gold, the added time and expense of nailing down the chamber volume to a perfect, repeatable figure within .1cc across all four jugs is really gilding the lily. It has taken me as long as a week to match a set of heads to 0.1cc, whereas getting them within 1.0cc ( plus or minus 0.5cc) is pretty easy.
Normally, when making small adjustments to your chamber volume you do so by sinking one of the valves. If you’ve got a lot of volume to make up, you sink the intake, but never very much. You want the intake valve to keep its head up. On the other hand, the exhaust valve can be down in a well and still work, thanks to the pressure during the exhaust cycle. But adjusting valve depth is not a trivial task. You need a set of stones and the tools and the skills. And you must be accustomed to working to very close tolerances. Go a bit too far and you’ll end up having to do the other three combustion chambers over again, to match your mistake.
You’ll encounter the same difficulties when you try to keep your deck height to some small value. Deck height determination starts before the engine is assembled. You need to determine the precise center-to-center length of each rod . . . they vary according to how the big end is honed . . . and on each piston, the distance between the wrist-pin bore and the head of the piston. You also must measure each of your cylinders using a surface plate and surface gauge. You do your first pre-assembly by matching ‘short’ rods to ‘long’ pistons in an effort to make all of equal length but you don’t really know anything until the crankcase is torqued and the cylinders seated with a dummy head, a plate with spacers that allows you to torque the cylinder studs to spec. Deck height is measured through a hole in the plate.
The trick here is to arrive at a consistent height for all of the cylinders while you maintain the same deck height. It is virtually impossible to do this without having a lathe, since the final dimension is usually achieved by shaving a few thou off the heads of the ‘fat’ pistons, but you can shuffle components to arrive at the best average.
Deck height is the most critical of your volumetric balance dimensions because a small dimensional change results in a large change in volume.
If you’re building a plain vanilla engine, measure everything but do not attempt to balance chamber volume until you’ve done at least the first pre-assembly to determine deck-height. Often times you’ll be able to balance a big chamber with a small deck-height and arrive at a good average volume across all four jugs.
A key point to keep in mind is that your engine will run with some amazing imbalances in both mass and volume, but it does so at an equally amazing cost. An out of balance engine is destined to live a short, expensive life whereas one that is balanced with respect to both mass and volume uses less fuel to produce the same power and has a useful life considerably longer than it’s mass-produced cousins. And these are improvements that are built-in for the life of the engine, repaying their cost many times over.
VW - Cleaning Tinware
Cleaning steel parts is a snap compared to aluminum or magnesium alloys. Just boil it. With lye. Not only will it remove all of the grease, it also takes off the paint and much of the rust.
A 55 gal oil drum cut in half and fitted with a lid makes a good parts boiler, a kind of poor-man’s hot tank. Or cold tank, although things happen more quickly when the stuff is hot. Provided with a pump, blower and a bit of plumbing, you can do a fine job of heating it using old lubricating oil. (If you’re interesting in foundry work, the same burner assembly provides enough heat to melt a good-sized crucible of aluminum in about twenty minutes.) The importance of the tin-ware is often overlooked. If you’re doing an overhaul you may find my previous post ‘Easy Tin’ of value.
A 55 gal oil drum cut in half and fitted with a lid makes a good parts boiler, a kind of poor-man’s hot tank. Or cold tank, although things happen more quickly when the stuff is hot. Provided with a pump, blower and a bit of plumbing, you can do a fine job of heating it using old lubricating oil. (If you’re interesting in foundry work, the same burner assembly provides enough heat to melt a good-sized crucible of aluminum in about twenty minutes.) The importance of the tin-ware is often overlooked. If you’re doing an overhaul you may find my previous post ‘Easy Tin’ of value.
VW - Compression Ratio
How to Adjusting Deck Height and Compression Ratio
The use of rebuilt heads poses a problem for the unwary. Wanna not blow up your engine? Read on!
Doing the Numbers
We need to start with some definitions. The manuals differ on how they label things so for now, use the following:
Compression ratio is determined by dividing the total volume (V sub t) by the swept volume (V sub s).
Total volume is the sum of the chamber volume (V sub c), the deck volume (V sub d) and the swept volume (V sub s)
Chamber volume is not calculated, it is measured directly. (The Factory Workshop Manual offers a good explanation of the procedure.) For your basic overhaul you don’t need a very precise number but you must know the approximate chamber volume. An easy way to do this is to obtain a fat 50cc syringe from a veterinary or pharmacy, level the head with the valves and spark plug installed, then fill the chamber with water. Do it several times and average the results. Do it for all four chambers. When you calculate your compression ratio, use the smallest of the four chambers for chamber volume (V sub c).
If the chambers are larger than 50cc use a marble to take up some of the space. Determine the marble’s volume by dropping it into the half-filled syringe. It’s volume equals its displacement. (Eureka!) Use the same marble(s) in all four chambers and don’t forget to add its volume to the reading off the syringe.
Swept volume and deck volume are calculated using the formula: 0.785 x bore diameter squared x stroke
Stroke is 69mm for a stock engine. If you’re rebuilding a 36hp, use 64mm. If your engine has a stroker crank, use that dimension. But don’t guess.
To calculate deck height, use the same formula but substitute deck height for stroke. Bore diameter is whatever pistons you are using. A stock 1600cc engine uses 85.5mm jugs.
Use millimeters for all of your calculations. 1mm is approximately equal to .0394 inches. Measure your deck height then convert it to millimeters by dividing the inch dimension by .0394
Since you’ll be using millimeters for your calculations, the answer will be in cubic millimeters. To convert to cubic centimeters, shove the decimal three places to the left.
For Example...
To illustrate, let me walk through the calculations using real data and real heads, a pair of rebuilts I have here in the shop.
The engine is a stock 1600cc dual-port. The jugs are 85.5mm in diameter, the crankshaft has a measured throw of 34.5 (i.e., a stroke of 69mm).
Since we use that same diameter when we calculate deck volume, let’s run the numbers and get it out of the way. 85.5 x 85.5 (that is, the diameter squared) equals 7,310.25. Now we multiply that by 0.785 (that is, the pi factor) to get 5,738.546. We will use that value to compute both swept volume and deck volume.
5,738.546 times the 69mm stroke gives us 395,959.6913 as our swept volume.
The deck height measured .065" Dividing that by .0394" gives us 1.649mm.
5,738.546 times the 1.649mm deck height gives us 9,462.86.
The combustion chamber measured 43.5 cubic centimeters so lets convert the other factors to cubic centimeters before we add them together.
395,959.6913 becomes.......... 396.0cc (rounded) Swept
9,462.86 becomes....................... 9.5cc (rounded) Deck
and our chamber volume was.... 43.5cc (averaged) Chamber
Which gives us a total volume of...449.0 Total Volume
(Note: Engine displacement is based on swept volume rather than total volume. Four times 396cc equals 1584, the displacement of a ‘1600' engine.)
Dividing the total volume by the sum of the chamber and deck volume gives us 449.0 divided by 53.0 or 8.346... which is our compression ratio. And that is too high.
A Bit of Background
This particular engine is in the shop because it blew a piston . . . hole about as big as a quarter, right through the top of the thing. And it was just overhauled, too.
How could such a thing happen? Easy. Kid talks his mom into buying him a VW. It’s a total POS but he’s convinced he can fix it up. Following the advice of the local guru, first thing the kid does is buy a pair of rebuilt heads and slap them on without bothering to check anything. But one of the heads had been flycut. Flycutting reduces the chamber volume, which raises the compression ratio. A combination of hot weather, a heavy foot, low octane gas and a high compression ratio lead to detonation. Naturally, the kid kept on driving. And of course he had to really keep his foot in it to get over them hills with only three cylinders.
The second jug blew a few minutes after the first, which convinced him to turn around and head for home. Amazingly, he managed to make it home on the two surviving cylinders. Of course, it cost him an engine. He now drives his mom’s Toyota and rails against veedubs as ‘nothing but junk.’ His mom, no dummy, won’t even let him check the air in the tires :-)
So you run the numbers and they say the compression ratio will be too high. What do we do now?
We put spacers under the cylinders, that’s what. Of course, we need to know how thick a spacer we should use but that turns out to be pretty simple, we merely turn the equation around.
More Numbers
With a total volume of 449cc, what volume chamber-plus-deck will give us 7.3:1?
To find out, just divide 449 by 7.3. You should get about sixty-one and half... 61.5cc’s.
And since you can’t change the chamber volume, lets get it out of the equation by subtracting it from the 61.5. 61.5 minus 43.5 equals 19.0cc, or about twice our original deck volume.
Since we measured the deck height as .065" the quick and dirty solution would be to double it by slapping a sixty-thou shim under the jugs and drive on. And it would work just fine, too. But quick & easy answers are often a bit too quick & easy. Remember, deck volume appears in both sides of the equation. If we increase our fixed volume . . . the volume of the deck plus the volume of the chamber . . . we have also increased our total volume. So before we dash off in all directions, lets run the numbers again. First, we’ll add 9.5cc to our total volume, making it 458.5 cc’s. Now we add 9.5cc’s to our fixed volume, making it 62.5cc. Now divide 458.5 by 62.5 and see what you get.
7.336?
Ah ha, said the mechanic. Ah ha indeed! Close enough.
(But what if the numbers had been off? In that case simply do the calculations over again, increasing deck height by ten thou (.25mm) each time until your compression ratio drops below 7.5 to 1.)
Okay, in this case the numbers worked out close enough. And a sixty-thou spacer is a standard item, if you call around. But be careful. Everyone carries tens, twenties and forties. They’ll tell you to stack them up to make a sixty but don’t do it, you want as few surfaces as possible. The good shops carry shims all the way up to ninty thou (.090") in increments of ten thou (.010") and only charge about eight bucks a set. cheep.
(Before posting this article I called around to verify availability and price of cylinder shims. A couple of outfits only carried the thinner three sizes, insisted it was okay to simply ‘stack ‘em up’ to get whatever thickness was required. The better shops carried the full range. Johnny’s Speed & Chrome sez stackemup, Mark Stephens’ shop was the other sort . . . “We got stock, ten thru ninety. Or we can polish you up a set.” I like their attitude.)
The range of available spacers brings up another point. The use of cylinder shims or spacers is the normal procedure used to adjust the compression ratio of a rebuilt engine and all of the better after-market suppliers keep the standard sizes on the shelf, ready for immediate delivery. But if you want a size that isn’t in stock, the price goes up dramatically since someone will have to chuck a set of spacers in a surface grinder and bring them down to the size you need. This can cost up to forty dollars.
So let’s say your numbers tell you to use a spacer exactly .035" thick. Unless you’re out to win a race, don’t do it. Order a set of forties and drive on. But don’t stack up your shims. It increases the risk of leaks.
The rule here is to opt for the next larger size of standard shims that will give you the compression ratio you need. And it’s handy to know that the paper gasket in the standard overhaul kit is about .008" thick. I don’t use gaskets on my engines since I prefer a metal-to-metal joint between the cylinders and crankcase, but in a special case with stock jugs, I might use them.
Sermonette
Stock Volkswagen engines (for gasoline) have used compression ratios as low as 5.8:1 and as high as 8.5:1. Given today’s gasoline, the wiser course is to err on the low side, with a compression ratio of no more than 7.5:1, and a whole lot less if you buy your gas at a Pemex station. (Air cooled engines for the domestic Mexican market are fitted with dished pistons that give a CR of 6.6:1, reflecting the low octane rating of Pemex regular gasoline.)
Rebuilt parts are liable to vary wildly from the dimension of stock, original Volkswagen equipment. When someone sez a part is ‘stock’ it don’t mean a thing until you clap a caliper on it and see for yourself that it falls within stock specs.
In an earlier series of posts I wrote about filter/pump adaptors that were machined about .006" undersize, rendering them useless. On returning the part to the store I demonstrated that it could not work as intended. Later I visited the same store and saw the same filter-pump in the showcase, waiting for the next sucker to come along.
When it comes to parts, rebuilt or new, it’s insane to trust the veracity of the guy behind the parts counter. A few simple tests and measurements not only eliminates confusion it usually results in a better engine.
The use of rebuilt heads poses a problem for the unwary. Wanna not blow up your engine? Read on!
Doing the Numbers
We need to start with some definitions. The manuals differ on how they label things so for now, use the following:
Compression ratio is determined by dividing the total volume (V sub t) by the swept volume (V sub s).
Total volume is the sum of the chamber volume (V sub c), the deck volume (V sub d) and the swept volume (V sub s)
Chamber volume is not calculated, it is measured directly. (The Factory Workshop Manual offers a good explanation of the procedure.) For your basic overhaul you don’t need a very precise number but you must know the approximate chamber volume. An easy way to do this is to obtain a fat 50cc syringe from a veterinary or pharmacy, level the head with the valves and spark plug installed, then fill the chamber with water. Do it several times and average the results. Do it for all four chambers. When you calculate your compression ratio, use the smallest of the four chambers for chamber volume (V sub c).
If the chambers are larger than 50cc use a marble to take up some of the space. Determine the marble’s volume by dropping it into the half-filled syringe. It’s volume equals its displacement. (Eureka!) Use the same marble(s) in all four chambers and don’t forget to add its volume to the reading off the syringe.
Swept volume and deck volume are calculated using the formula: 0.785 x bore diameter squared x stroke
Stroke is 69mm for a stock engine. If you’re rebuilding a 36hp, use 64mm. If your engine has a stroker crank, use that dimension. But don’t guess.
To calculate deck height, use the same formula but substitute deck height for stroke. Bore diameter is whatever pistons you are using. A stock 1600cc engine uses 85.5mm jugs.
Use millimeters for all of your calculations. 1mm is approximately equal to .0394 inches. Measure your deck height then convert it to millimeters by dividing the inch dimension by .0394
Since you’ll be using millimeters for your calculations, the answer will be in cubic millimeters. To convert to cubic centimeters, shove the decimal three places to the left.
For Example...
To illustrate, let me walk through the calculations using real data and real heads, a pair of rebuilts I have here in the shop.
The engine is a stock 1600cc dual-port. The jugs are 85.5mm in diameter, the crankshaft has a measured throw of 34.5 (i.e., a stroke of 69mm).
Since we use that same diameter when we calculate deck volume, let’s run the numbers and get it out of the way. 85.5 x 85.5 (that is, the diameter squared) equals 7,310.25. Now we multiply that by 0.785 (that is, the pi factor) to get 5,738.546. We will use that value to compute both swept volume and deck volume.
5,738.546 times the 69mm stroke gives us 395,959.6913 as our swept volume.
The deck height measured .065" Dividing that by .0394" gives us 1.649mm.
5,738.546 times the 1.649mm deck height gives us 9,462.86.
The combustion chamber measured 43.5 cubic centimeters so lets convert the other factors to cubic centimeters before we add them together.
395,959.6913 becomes.......... 396.0cc (rounded) Swept
9,462.86 becomes....................... 9.5cc (rounded) Deck
and our chamber volume was.... 43.5cc (averaged) Chamber
Which gives us a total volume of...449.0 Total Volume
(Note: Engine displacement is based on swept volume rather than total volume. Four times 396cc equals 1584, the displacement of a ‘1600' engine.)
Dividing the total volume by the sum of the chamber and deck volume gives us 449.0 divided by 53.0 or 8.346... which is our compression ratio. And that is too high.
A Bit of Background
This particular engine is in the shop because it blew a piston . . . hole about as big as a quarter, right through the top of the thing. And it was just overhauled, too.
How could such a thing happen? Easy. Kid talks his mom into buying him a VW. It’s a total POS but he’s convinced he can fix it up. Following the advice of the local guru, first thing the kid does is buy a pair of rebuilt heads and slap them on without bothering to check anything. But one of the heads had been flycut. Flycutting reduces the chamber volume, which raises the compression ratio. A combination of hot weather, a heavy foot, low octane gas and a high compression ratio lead to detonation. Naturally, the kid kept on driving. And of course he had to really keep his foot in it to get over them hills with only three cylinders.
The second jug blew a few minutes after the first, which convinced him to turn around and head for home. Amazingly, he managed to make it home on the two surviving cylinders. Of course, it cost him an engine. He now drives his mom’s Toyota and rails against veedubs as ‘nothing but junk.’ His mom, no dummy, won’t even let him check the air in the tires :-)
So you run the numbers and they say the compression ratio will be too high. What do we do now?
We put spacers under the cylinders, that’s what. Of course, we need to know how thick a spacer we should use but that turns out to be pretty simple, we merely turn the equation around.
More Numbers
With a total volume of 449cc, what volume chamber-plus-deck will give us 7.3:1?
To find out, just divide 449 by 7.3. You should get about sixty-one and half... 61.5cc’s.
And since you can’t change the chamber volume, lets get it out of the equation by subtracting it from the 61.5. 61.5 minus 43.5 equals 19.0cc, or about twice our original deck volume.
Since we measured the deck height as .065" the quick and dirty solution would be to double it by slapping a sixty-thou shim under the jugs and drive on. And it would work just fine, too. But quick & easy answers are often a bit too quick & easy. Remember, deck volume appears in both sides of the equation. If we increase our fixed volume . . . the volume of the deck plus the volume of the chamber . . . we have also increased our total volume. So before we dash off in all directions, lets run the numbers again. First, we’ll add 9.5cc to our total volume, making it 458.5 cc’s. Now we add 9.5cc’s to our fixed volume, making it 62.5cc. Now divide 458.5 by 62.5 and see what you get.
7.336?
Ah ha, said the mechanic. Ah ha indeed! Close enough.
(But what if the numbers had been off? In that case simply do the calculations over again, increasing deck height by ten thou (.25mm) each time until your compression ratio drops below 7.5 to 1.)
Okay, in this case the numbers worked out close enough. And a sixty-thou spacer is a standard item, if you call around. But be careful. Everyone carries tens, twenties and forties. They’ll tell you to stack them up to make a sixty but don’t do it, you want as few surfaces as possible. The good shops carry shims all the way up to ninty thou (.090") in increments of ten thou (.010") and only charge about eight bucks a set. cheep.
(Before posting this article I called around to verify availability and price of cylinder shims. A couple of outfits only carried the thinner three sizes, insisted it was okay to simply ‘stack ‘em up’ to get whatever thickness was required. The better shops carried the full range. Johnny’s Speed & Chrome sez stackemup, Mark Stephens’ shop was the other sort . . . “We got stock, ten thru ninety. Or we can polish you up a set.” I like their attitude.)
The range of available spacers brings up another point. The use of cylinder shims or spacers is the normal procedure used to adjust the compression ratio of a rebuilt engine and all of the better after-market suppliers keep the standard sizes on the shelf, ready for immediate delivery. But if you want a size that isn’t in stock, the price goes up dramatically since someone will have to chuck a set of spacers in a surface grinder and bring them down to the size you need. This can cost up to forty dollars.
So let’s say your numbers tell you to use a spacer exactly .035" thick. Unless you’re out to win a race, don’t do it. Order a set of forties and drive on. But don’t stack up your shims. It increases the risk of leaks.
The rule here is to opt for the next larger size of standard shims that will give you the compression ratio you need. And it’s handy to know that the paper gasket in the standard overhaul kit is about .008" thick. I don’t use gaskets on my engines since I prefer a metal-to-metal joint between the cylinders and crankcase, but in a special case with stock jugs, I might use them.
Sermonette
Stock Volkswagen engines (for gasoline) have used compression ratios as low as 5.8:1 and as high as 8.5:1. Given today’s gasoline, the wiser course is to err on the low side, with a compression ratio of no more than 7.5:1, and a whole lot less if you buy your gas at a Pemex station. (Air cooled engines for the domestic Mexican market are fitted with dished pistons that give a CR of 6.6:1, reflecting the low octane rating of Pemex regular gasoline.)
Rebuilt parts are liable to vary wildly from the dimension of stock, original Volkswagen equipment. When someone sez a part is ‘stock’ it don’t mean a thing until you clap a caliper on it and see for yourself that it falls within stock specs.
In an earlier series of posts I wrote about filter/pump adaptors that were machined about .006" undersize, rendering them useless. On returning the part to the store I demonstrated that it could not work as intended. Later I visited the same store and saw the same filter-pump in the showcase, waiting for the next sucker to come along.
When it comes to parts, rebuilt or new, it’s insane to trust the veracity of the guy behind the parts counter. A few simple tests and measurements not only eliminates confusion it usually results in a better engine.
VW - Engine Break-In
I think I need to re-check the head-torque before I run it... Is there a preferred pattern to loosening the nuts?
If the engine has never run, you’re probably safe in unbolting it willy-nilly, but I tend to work form the outside in, top to the bottom. Make everything finger-loose. Check for ‘tight’ nuts. Don’t disturb the washers; there should be sealant under them. With everything backed off, use MEK or Loc-tite’s ‘Klean-n’-Prime’ on the thread between the nut and the washer (you’ll need the little nozzle, or a laboratory spray bottle, or Q-tips, or... I use one of those lab bottles).
If you really can’t get in there, take off the nut so you can get at the stud. If you got tight nuts, replace them or chase them.
When you like the way things fit, put a dot of medium strength Loc-tite on the primed thread right up by the washer, run the nuts up finger tight plus a twist ‘o the wrist, start sneaking up on the torque. I resent my clicker three or four times, starting at about 12, ending up with 25, 26, or 27 ft/lbs, depending on temperature, type of crankcase, direction of the wind... :-) If it’s an early case with thick studs, use 23 ft/lb. Later cases with stud-inserts and the small (8mm) studs have a lower torquing spec, but never exceed 18 ft/lb. After retorquing the new engine, let it sit over night then check the torque again before you close it up. Just check for the last value; you’re looking for ‘lazy’ studs that have elogated as a result of torquing.
To me, First Fire doesn’t really count as part of the break-in, it’s merely the final step in the assembly. I get it started, pick it up to about a thousand rpm, let it run until it has a certain ‘hot’ smell, never letting the speed settle, up and down a little, senses very busy. Mebbe three minutes, max. Oil is 10W-Something; thin. Then I drain it out into a clean container, go off and think about things for a while. Slowly decant the flushing oil, drain the last little bit into a glass dish, slosh it with MEK like a placer miner looking for gold.
I’ve just cleaned the inside of the newly assembled engine. It really isn’t a part of the break-in cycle. Back when I was building a lot of engines, I might even use the same flushing oil on two engines, or put the same oil back in the engine, if no residue was found (but I usually throw it away; about four bucks. Cheep).
After it’s flushed, I re-check the valves and timing; anything that might have kicked loose; anything I might have overlooked. (Yeah, me too.) Then I refill it, fire it up, warm it up . . . never takes long with a new engine . . . bring it up to about 2,500, hunt around that speed range while I’m crawling all over the thing. It’s on the test stand, I’m not wearing ear muffs. I’ve got all the sensors cranked up to nine; smell, sight, hearing, hearing, hearing, touch. I overload in about fifteen minutes, have to shut it down and think about it. I might run it a little more to get more data on a particular thing but most often drain it, let it cool down. You always throw away this oil; it’s your first break-in cycle. Check it for chunkies of course. You’re real interested in any drips at this stage. There shouldn’t be a single one so if you see one it usually means the thing goes back into the shop, gets torn down. This is about the worst thing that can happen. And it does, but no oftener than once in every hundred engines or so. And yes, it’s usually my fault. Just plain damn stupidity or carelessness, like letting my cuff drag across the parting line just as I’m closing the case; dumb stuff. All that work ruined. But I’m getting better at it :-)
I usually keep an engine on the test stand until the rings are well seated and the temperature comes down; about six hours, on average. Then too, I tend to build ‘tight’ engines; you have to be careful breaking them in, giving them plenty of time to cool down between runs, keeping the oil changed even with the filter installed.
After a couple of hours you and the engine are pretty well acquainted; they’re all a little different. You’ve got the carb(s) tweaked down to the fine numbers, the thing will start on the first revolution of the crank, the case is dry, the blow-back is next to nothing and it’s starting to develop its own unique sound that will allow me to identify it years from now, assuming the muffler and intake are the same.
An apparently mindless part of breaking in an engine is seating the rings. We call it Bumps & Grinds. You slowly open the throttle to about 2500 then drop it, just let it shut. Anyone hearing you would think you’re just playing with the poor thing, up and down, loud then quiet. You’ll be wearing your ear muffs by then; you’re breaking it in, not checking it out. You never do any of these things for very long; mebbe 20 minutes at the outside, more like fifteen, then shut it down and go do something else; let it cool off. If you’re doing more than one engine you’ll have to dismount it hot, put it back on its scooter, put the next customer in the chair. (I’ve done as many as five engines for one customer, all as a series. Five is mebbe one too many for one man at a time.)
You keep records. If you’re using a test stand you’ll usually have a Hobbs meter; a kind of clock. If not, just your watch and and a note book. Doing one engine, you can’t get too confused.
The engine will start flattening out its curves after five or six hours of running. Fuel consumption will have dropped down to some steady figure, as will the temperatures. Oil pressure will have come up. You’ll want to verify that with a wet & dry compression check and a leak-down test, if you’ve got the harness. You will have to take a last look at the valves . . . unless you’re running hydraulics.
Breaking in an engine with hydraulic lifters is a little different than with solids, first because the valves probably won’t tell you anything at all. They’ll always be running a perfect zero-lash. But hydraulics in a freshly machined magnesium crankcase can generate a bit more ‘mud’ than with solids, or even hydraulics in an aluminum crankcase. So you take it easier at first, accumulating about 45 minutes of run-time before you start seating in the rings. Understand, the engine has never been allowed to sit about at an idle. Breaking in an engine means wearing-in an engine; it is a kind of controlled friction. But the mud does bad things to the minute clearances of hydraulic lifters, which are having an especially hard time because the engine is running hotter than it ever will again. So you do more short runs and longer cooling-off periods when you break-in a Type I fitted with hydraulics. That is, during the first two to three hours. After that, there doesn’t seem to be any difference, except the hydraulic engine will run quieter. And a little more efficiently, but you won’t see that until you’ve got mebbe six hours on it, by which time it probably will have been installed in a vehicle and roared off down the road.
Air-cooled engines have pretty loose tolerances compared to their water-cooled cousins. And with the inherent rev-limiters built in the the VW carburetion, cam and valves, you could run a factory-built VW right out of the box and not have any problems. That was then. No telling what kind of an engine you’ll wind up with nowadays. It’s best to pretend it’s a custom-assembled one-off design and break it in accordingly, taking lots of time to correct any problems, let the thing cool between runs (and I mean at least an hour), change the oil at least twice in the first six hours, and doing anything else you can think of to ease the birthing pains.
The complete wear-in cycle continues for about a hundred hours, after which the engine’s curves will stay substantially the same for the next thousand hours of engine operation. (Ed.Note: In a vehicle.) Understand, the engine is still wearing, still experiencing friction. But now it is wearing-out, not in. The wear during its service life will be very uniform and consistently small, but after a thousand hours or so you will see the first signs of terminal wear from the valves, the weak links in the VW system. The lower end should be good for at least 1,500 hours and will probably run 2,000 without a whit of trouble (say, 100,000 miles) assuming you’re running a full-flow filter. Beyond that, it will depend on if you’ve got a shaft seal, how well you’ve done your maintenance, your particular driving habits, and the vehicle in which the engine is installed, with early buses providing about half the service life of a sedan engine, later buses falling somewhere between the two.
Given the fact this is your first engine, I’m really looking forward to you having a successful installation. Please keep the list up to date on your efforts. Although you may not believe it, your success will cause of at least three other subscribers to take the plunge. It isn’t just one engine you’re bringing to life here but four.
If the engine has never run, you’re probably safe in unbolting it willy-nilly, but I tend to work form the outside in, top to the bottom. Make everything finger-loose. Check for ‘tight’ nuts. Don’t disturb the washers; there should be sealant under them. With everything backed off, use MEK or Loc-tite’s ‘Klean-n’-Prime’ on the thread between the nut and the washer (you’ll need the little nozzle, or a laboratory spray bottle, or Q-tips, or... I use one of those lab bottles).
If you really can’t get in there, take off the nut so you can get at the stud. If you got tight nuts, replace them or chase them.
When you like the way things fit, put a dot of medium strength Loc-tite on the primed thread right up by the washer, run the nuts up finger tight plus a twist ‘o the wrist, start sneaking up on the torque. I resent my clicker three or four times, starting at about 12, ending up with 25, 26, or 27 ft/lbs, depending on temperature, type of crankcase, direction of the wind... :-) If it’s an early case with thick studs, use 23 ft/lb. Later cases with stud-inserts and the small (8mm) studs have a lower torquing spec, but never exceed 18 ft/lb. After retorquing the new engine, let it sit over night then check the torque again before you close it up. Just check for the last value; you’re looking for ‘lazy’ studs that have elogated as a result of torquing.
To me, First Fire doesn’t really count as part of the break-in, it’s merely the final step in the assembly. I get it started, pick it up to about a thousand rpm, let it run until it has a certain ‘hot’ smell, never letting the speed settle, up and down a little, senses very busy. Mebbe three minutes, max. Oil is 10W-Something; thin. Then I drain it out into a clean container, go off and think about things for a while. Slowly decant the flushing oil, drain the last little bit into a glass dish, slosh it with MEK like a placer miner looking for gold.
I’ve just cleaned the inside of the newly assembled engine. It really isn’t a part of the break-in cycle. Back when I was building a lot of engines, I might even use the same flushing oil on two engines, or put the same oil back in the engine, if no residue was found (but I usually throw it away; about four bucks. Cheep).
After it’s flushed, I re-check the valves and timing; anything that might have kicked loose; anything I might have overlooked. (Yeah, me too.) Then I refill it, fire it up, warm it up . . . never takes long with a new engine . . . bring it up to about 2,500, hunt around that speed range while I’m crawling all over the thing. It’s on the test stand, I’m not wearing ear muffs. I’ve got all the sensors cranked up to nine; smell, sight, hearing, hearing, hearing, touch. I overload in about fifteen minutes, have to shut it down and think about it. I might run it a little more to get more data on a particular thing but most often drain it, let it cool down. You always throw away this oil; it’s your first break-in cycle. Check it for chunkies of course. You’re real interested in any drips at this stage. There shouldn’t be a single one so if you see one it usually means the thing goes back into the shop, gets torn down. This is about the worst thing that can happen. And it does, but no oftener than once in every hundred engines or so. And yes, it’s usually my fault. Just plain damn stupidity or carelessness, like letting my cuff drag across the parting line just as I’m closing the case; dumb stuff. All that work ruined. But I’m getting better at it :-)
I usually keep an engine on the test stand until the rings are well seated and the temperature comes down; about six hours, on average. Then too, I tend to build ‘tight’ engines; you have to be careful breaking them in, giving them plenty of time to cool down between runs, keeping the oil changed even with the filter installed.
After a couple of hours you and the engine are pretty well acquainted; they’re all a little different. You’ve got the carb(s) tweaked down to the fine numbers, the thing will start on the first revolution of the crank, the case is dry, the blow-back is next to nothing and it’s starting to develop its own unique sound that will allow me to identify it years from now, assuming the muffler and intake are the same.
An apparently mindless part of breaking in an engine is seating the rings. We call it Bumps & Grinds. You slowly open the throttle to about 2500 then drop it, just let it shut. Anyone hearing you would think you’re just playing with the poor thing, up and down, loud then quiet. You’ll be wearing your ear muffs by then; you’re breaking it in, not checking it out. You never do any of these things for very long; mebbe 20 minutes at the outside, more like fifteen, then shut it down and go do something else; let it cool off. If you’re doing more than one engine you’ll have to dismount it hot, put it back on its scooter, put the next customer in the chair. (I’ve done as many as five engines for one customer, all as a series. Five is mebbe one too many for one man at a time.)
You keep records. If you’re using a test stand you’ll usually have a Hobbs meter; a kind of clock. If not, just your watch and and a note book. Doing one engine, you can’t get too confused.
The engine will start flattening out its curves after five or six hours of running. Fuel consumption will have dropped down to some steady figure, as will the temperatures. Oil pressure will have come up. You’ll want to verify that with a wet & dry compression check and a leak-down test, if you’ve got the harness. You will have to take a last look at the valves . . . unless you’re running hydraulics.
Breaking in an engine with hydraulic lifters is a little different than with solids, first because the valves probably won’t tell you anything at all. They’ll always be running a perfect zero-lash. But hydraulics in a freshly machined magnesium crankcase can generate a bit more ‘mud’ than with solids, or even hydraulics in an aluminum crankcase. So you take it easier at first, accumulating about 45 minutes of run-time before you start seating in the rings. Understand, the engine has never been allowed to sit about at an idle. Breaking in an engine means wearing-in an engine; it is a kind of controlled friction. But the mud does bad things to the minute clearances of hydraulic lifters, which are having an especially hard time because the engine is running hotter than it ever will again. So you do more short runs and longer cooling-off periods when you break-in a Type I fitted with hydraulics. That is, during the first two to three hours. After that, there doesn’t seem to be any difference, except the hydraulic engine will run quieter. And a little more efficiently, but you won’t see that until you’ve got mebbe six hours on it, by which time it probably will have been installed in a vehicle and roared off down the road.
Air-cooled engines have pretty loose tolerances compared to their water-cooled cousins. And with the inherent rev-limiters built in the the VW carburetion, cam and valves, you could run a factory-built VW right out of the box and not have any problems. That was then. No telling what kind of an engine you’ll wind up with nowadays. It’s best to pretend it’s a custom-assembled one-off design and break it in accordingly, taking lots of time to correct any problems, let the thing cool between runs (and I mean at least an hour), change the oil at least twice in the first six hours, and doing anything else you can think of to ease the birthing pains.
The complete wear-in cycle continues for about a hundred hours, after which the engine’s curves will stay substantially the same for the next thousand hours of engine operation. (Ed.Note: In a vehicle.) Understand, the engine is still wearing, still experiencing friction. But now it is wearing-out, not in. The wear during its service life will be very uniform and consistently small, but after a thousand hours or so you will see the first signs of terminal wear from the valves, the weak links in the VW system. The lower end should be good for at least 1,500 hours and will probably run 2,000 without a whit of trouble (say, 100,000 miles) assuming you’re running a full-flow filter. Beyond that, it will depend on if you’ve got a shaft seal, how well you’ve done your maintenance, your particular driving habits, and the vehicle in which the engine is installed, with early buses providing about half the service life of a sedan engine, later buses falling somewhere between the two.
Given the fact this is your first engine, I’m really looking forward to you having a successful installation. Please keep the list up to date on your efforts. Although you may not believe it, your success will cause of at least three other subscribers to take the plunge. It isn’t just one engine you’re bringing to life here but four.
VW - Bug Eats Lexus! (News at eleven)
Friday. Had to run some errands in town. Took the baja instead of the bike. Guy talking on a cell phone decided he wanted to occupy my lane. With me in it. Lexus. No turn signal, he just decided to move over. I stayed alongside, smiled. Went to cut me off, apparently willing to play bumper tag with a baja that has crash bars on the sides as well as front, back, top, bottom and a few places in between.
We were up to about 60 on a 4-lane city street, intersection coming up, light is green and I'm signalling for the turn. The Yuppie Prince, not in a turning lane, is being forced to Plan Ahead for the first time in years. He decides to floor it. After all, $50,000 vs a baja bug?
I gave the accelerator a gentle touch of toe and at that precise, wonderful moment, I ate the fan belt! Engine immediately went to about nine thousand rpm, slams me back into the seat like something out of ‘Road Warrior’ breathing nitrous. Lights come on, horn goes off, speedo winds up to something seriously silly as I shoot through the intersection on the tail-end of the green, hang a left, coast into Carrow’s parking lot leaving the Yuppie Prince in his $50,000 cage to sort it out with Deputy Dawg, who he almost creamed diving into the intersection on the yellow. The Deputy was still writing in his little book by the time I got the spare belt installed.
Sometimes God frowns. But sometimes She smiles, too.
We were up to about 60 on a 4-lane city street, intersection coming up, light is green and I'm signalling for the turn. The Yuppie Prince, not in a turning lane, is being forced to Plan Ahead for the first time in years. He decides to floor it. After all, $50,000 vs a baja bug?
I gave the accelerator a gentle touch of toe and at that precise, wonderful moment, I ate the fan belt! Engine immediately went to about nine thousand rpm, slams me back into the seat like something out of ‘Road Warrior’ breathing nitrous. Lights come on, horn goes off, speedo winds up to something seriously silly as I shoot through the intersection on the tail-end of the green, hang a left, coast into Carrow’s parking lot leaving the Yuppie Prince in his $50,000 cage to sort it out with Deputy Dawg, who he almost creamed diving into the intersection on the yellow. The Deputy was still writing in his little book by the time I got the spare belt installed.
Sometimes God frowns. But sometimes She smiles, too.
AV - Tail Flapper Failure
Wednesday 19 May 2004
My tail stopped flapping yesterday. I don't know exactly when. It was okay when I checked it around nine a.m. I was in & out of the shop several times during the day and could hear the motor but I didn't look to see if it was still flapping. When I checked it about ten p.m. this evening the motor was still running but sounded different. The tail was no longer flapping.
Obvious case of Pilot Error.
The motor and its attached gear box is a high-quality item, purchased as new-surplus for just a couple of bucks because the output shaft is metric - - 12mm instead of an honest half-inch. Ami-Ricans go sorta silly when you mention metric, turn their nose up even if it's quality goods, which this unit is. What failed was the grub screw in the disk I've attached to the output shaft.
The disk is a hunka half-inch aluminum tooling plate, about 2.25" diameter. It's turned down to a thickness of .125" except for the hub which is fitted with a #6 set screw and reamed to accept the gear-motor's 12mm shaft, which has a flat for the set-screw. The disk is drilled with a series of .191" holes to accept an AN3 bolt. The holes are in a spiral, each one a precise tenth of an inch farther from the center of the disk, starting just outboard of the hub. I've been using the first hole to flap my tail-hinges. The first hole doesn't give a lot of motion, about three-quarters of an inch, in and out. But lots of power.
The tail I've been flapping isn't much to look at. About 10" wide, mebbe six deep. Just two hinges. Not covered or anything, just the hinge-line spar of 3/4" square wood with a piece of 1/8" ply glued to it, sticking out aft. I put a pound of lead on the plywood, a precise six inches from the center-line of the pivot point. Every time the tail flaps, it raises that pile of wheel weights about four and a half inches. It does that about 20 times a minute and has for the last forty days or so. Good little motor. Nice gear-box. Except for being metric, of course.
The hinges are made out of steel strapping; some junk I hauled home from a job site about a year ago. The strapping is mild steel, .020" thick by .625" wide. It's easy to bend yet surprisingly strong. I did the original calcs using 30kpsi but tear-out tests showed the metal is stronger than that; probably closer to 50kpsi. In theory, the stuff is strong enough to be used for hinges but it's so thin I guessed it would fail at the fastener. Or the thin section would cut through the pivot pin.
But guessing isn't knowing.
I made some C-shaped hinges. For the pivot pin I used AN393-47 clevis pins. That's a pin .191" in diameter and about an inch and a half long. I attached sets of hinges, usually in pairs, to three-quarter inch square stock - - hemlock or Douglas fir, whatever came to hand - - using nuts & bolts. Then I tore them apart using an hydrualic press, guesstimating the amount of force involved by the pressure on the cylinder at the moment of failure.
As a test of fasteners, it was a complete failure because the fasteners didn't. The strapping would tear out around the pivot pin or the wood would fail where it was attached to my puller. I fastened the hinges to the wood with AN3 nuts & bolts, with flat-head machine screws, even with crappy little 6-32 hardware (4 ea). All proved more durable than the material of the hinge.
Which is another way of saying my fasteners were too expensive. And too heavy.
Eventually I worked my way down to SEWING the hinges on using .025 SS safety wire, threaded through six or eight #40 holes. After twisting the wire tight I'd squirt the holes full of urethane glue then let it cure. To provide a bit of lateral support, I butted a scrap of 1/16" plywood about the size of my thumbnail up against the bent end of the hinge, used some more urethane glue. Now I started seeing some failure of the fastener... at about the same time the AN3 hinge-pin would start to tear out. The loads at that point was on the order of five hundred pounds, givertake.
Clevis pins aren't cheap. Actually, at about half a buck they're cheaper than dirt compared to most other aircraft certified hardware but they were also the strongest part of the hinge. Could I find a pin that worked as well but cost less? I donno. The only way to find out was to try different stuff.
I tried steel rod, nails and even clothes-line wire. At a pin diameter of about an eighth of an inch, with a mild steel pin, I began to see evidence of shearing in the pin along with the anticipated tear-out of the strapping. Load (per hinge) at that point was about 350 pounds.
Small diameter pins were something of a problem because they were difficult to secure. Using a nail as a sort of Po' Boy clevis pin, the head of the nail secures one end of the pin but you had to drill the other to accept a small Cotter key or safety wire in a Northrop loop. Eventually I struck upon using the Cotter key itself as the pin. BIG Cotter key... AN380-4-5. That's a Cotter key 1/8" in diameter and about an inch and a quarter long.
Worse-Case load for the elevator I had in mind was 540 pounds distributed across six hinges and I was seeing better than 300 pounds from a hinge that weighed about an ounce and cost less than a dime. It was kinda goofy and fragile looking but it was a surprisingly tough little hinge.
In addition to using the strapping as a pair of identical but overlapping ‘C's, I also experimented with L-shapes and even T-shapes, each of which offers some advantage, either through increasing the strength of the fastening to the wood or increasing the surface area where the pin bears upon the strapping.
I've never heard of anyone using steel strapping for hinges, nor sewing them to the spar. I tried to locate data on similar hinge arrangements and came up dry so I laid out a test program, made up the tail-flapper and started flapping. Of course, just flapping the thing really isn't much of a test. Nothing for UV exposure nor corrosion but I did run some vibration tests. Adding a small motor to the flapper and fastening a short length of steel eccentrically to the shaft gives you an Industrial Grade vibrator that wears out the hinge pins quick like a bunny, along with the motor :-)
The current flapper has been flapping since the afternoon of April third. About 1.3 million cycles. Two months would have given me about two million cycles. Earlier versions usually crapped out after ten days to two weeks due to excessive wear at the pivot point.
I've lubed the hinges with a variety of stuff. Right now I'm using dry tungsten disulfide powder, burnished into the surface of the Cotter key and the bores in which it rests. Seems to work pretty good. The lubricant seems to be the most critical factor although how accurately the samples are made also plays a role.
Anyone else out there trying to push back the darkness? Kevlar roving as the tension members in a fuselage using a Howe truss? Vacuum bagging leading-edge skins? If so, I'd be delighted to hear from you. But privately, please. I don't want to detract from the real purpose of rah.
-R.S.Hoover
My tail stopped flapping yesterday. I don't know exactly when. It was okay when I checked it around nine a.m. I was in & out of the shop several times during the day and could hear the motor but I didn't look to see if it was still flapping. When I checked it about ten p.m. this evening the motor was still running but sounded different. The tail was no longer flapping.
Obvious case of Pilot Error.
The motor and its attached gear box is a high-quality item, purchased as new-surplus for just a couple of bucks because the output shaft is metric - - 12mm instead of an honest half-inch. Ami-Ricans go sorta silly when you mention metric, turn their nose up even if it's quality goods, which this unit is. What failed was the grub screw in the disk I've attached to the output shaft.
The disk is a hunka half-inch aluminum tooling plate, about 2.25" diameter. It's turned down to a thickness of .125" except for the hub which is fitted with a #6 set screw and reamed to accept the gear-motor's 12mm shaft, which has a flat for the set-screw. The disk is drilled with a series of .191" holes to accept an AN3 bolt. The holes are in a spiral, each one a precise tenth of an inch farther from the center of the disk, starting just outboard of the hub. I've been using the first hole to flap my tail-hinges. The first hole doesn't give a lot of motion, about three-quarters of an inch, in and out. But lots of power.
The tail I've been flapping isn't much to look at. About 10" wide, mebbe six deep. Just two hinges. Not covered or anything, just the hinge-line spar of 3/4" square wood with a piece of 1/8" ply glued to it, sticking out aft. I put a pound of lead on the plywood, a precise six inches from the center-line of the pivot point. Every time the tail flaps, it raises that pile of wheel weights about four and a half inches. It does that about 20 times a minute and has for the last forty days or so. Good little motor. Nice gear-box. Except for being metric, of course.
The hinges are made out of steel strapping; some junk I hauled home from a job site about a year ago. The strapping is mild steel, .020" thick by .625" wide. It's easy to bend yet surprisingly strong. I did the original calcs using 30kpsi but tear-out tests showed the metal is stronger than that; probably closer to 50kpsi. In theory, the stuff is strong enough to be used for hinges but it's so thin I guessed it would fail at the fastener. Or the thin section would cut through the pivot pin.
But guessing isn't knowing.
I made some C-shaped hinges. For the pivot pin I used AN393-47 clevis pins. That's a pin .191" in diameter and about an inch and a half long. I attached sets of hinges, usually in pairs, to three-quarter inch square stock - - hemlock or Douglas fir, whatever came to hand - - using nuts & bolts. Then I tore them apart using an hydrualic press, guesstimating the amount of force involved by the pressure on the cylinder at the moment of failure.
As a test of fasteners, it was a complete failure because the fasteners didn't. The strapping would tear out around the pivot pin or the wood would fail where it was attached to my puller. I fastened the hinges to the wood with AN3 nuts & bolts, with flat-head machine screws, even with crappy little 6-32 hardware (4 ea). All proved more durable than the material of the hinge.
Which is another way of saying my fasteners were too expensive. And too heavy.
Eventually I worked my way down to SEWING the hinges on using .025 SS safety wire, threaded through six or eight #40 holes. After twisting the wire tight I'd squirt the holes full of urethane glue then let it cure. To provide a bit of lateral support, I butted a scrap of 1/16" plywood about the size of my thumbnail up against the bent end of the hinge, used some more urethane glue. Now I started seeing some failure of the fastener... at about the same time the AN3 hinge-pin would start to tear out. The loads at that point was on the order of five hundred pounds, givertake.
Clevis pins aren't cheap. Actually, at about half a buck they're cheaper than dirt compared to most other aircraft certified hardware but they were also the strongest part of the hinge. Could I find a pin that worked as well but cost less? I donno. The only way to find out was to try different stuff.
I tried steel rod, nails and even clothes-line wire. At a pin diameter of about an eighth of an inch, with a mild steel pin, I began to see evidence of shearing in the pin along with the anticipated tear-out of the strapping. Load (per hinge) at that point was about 350 pounds.
Small diameter pins were something of a problem because they were difficult to secure. Using a nail as a sort of Po' Boy clevis pin, the head of the nail secures one end of the pin but you had to drill the other to accept a small Cotter key or safety wire in a Northrop loop. Eventually I struck upon using the Cotter key itself as the pin. BIG Cotter key... AN380-4-5. That's a Cotter key 1/8" in diameter and about an inch and a quarter long.
Worse-Case load for the elevator I had in mind was 540 pounds distributed across six hinges and I was seeing better than 300 pounds from a hinge that weighed about an ounce and cost less than a dime. It was kinda goofy and fragile looking but it was a surprisingly tough little hinge.
In addition to using the strapping as a pair of identical but overlapping ‘C's, I also experimented with L-shapes and even T-shapes, each of which offers some advantage, either through increasing the strength of the fastening to the wood or increasing the surface area where the pin bears upon the strapping.
I've never heard of anyone using steel strapping for hinges, nor sewing them to the spar. I tried to locate data on similar hinge arrangements and came up dry so I laid out a test program, made up the tail-flapper and started flapping. Of course, just flapping the thing really isn't much of a test. Nothing for UV exposure nor corrosion but I did run some vibration tests. Adding a small motor to the flapper and fastening a short length of steel eccentrically to the shaft gives you an Industrial Grade vibrator that wears out the hinge pins quick like a bunny, along with the motor :-)
The current flapper has been flapping since the afternoon of April third. About 1.3 million cycles. Two months would have given me about two million cycles. Earlier versions usually crapped out after ten days to two weeks due to excessive wear at the pivot point.
I've lubed the hinges with a variety of stuff. Right now I'm using dry tungsten disulfide powder, burnished into the surface of the Cotter key and the bores in which it rests. Seems to work pretty good. The lubricant seems to be the most critical factor although how accurately the samples are made also plays a role.
Anyone else out there trying to push back the darkness? Kevlar roving as the tension members in a fuselage using a Howe truss? Vacuum bagging leading-edge skins? If so, I'd be delighted to hear from you. But privately, please. I don't want to detract from the real purpose of rah.
-R.S.Hoover
AV - How to Eat a DC-3
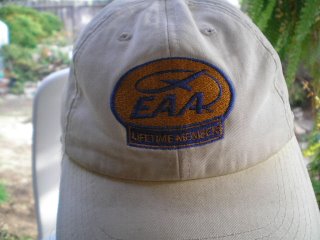
Actually, this is more about doing your laundry but you put something like that in the Subject Line on a post to R.A.H., you get lottsa strange mail. But just to keep things honest I will tell you how to eat a Gooney Bird. But in a minute, okay?
When I go up to Fla-Bob or Muroc I always wear my Catalina hat. Cheap looking thing and it doesn't really mean you been there but most guys who have, do. Gets dirty, though. All hats do, you wear them enough.
EAA sent me a Life Member hat. Seriously! Life member for thirty years, you get a hat. Comes in a box an everything. White. Can't touch it without leaving a mark. But I wear it now & then. Part of my Fly-in Costume. Too bad the EAA don't have fly-in's no more. (I'm too embarrassed to go to something called an 'airventure.')
Wear your EAA Life Member Hat to a fly-in, somebody always asks where you got it. "Salvation Army store." Or that I found it at a garage sale. Sometimes they offer to buy it. I whip it off with a grin, "Sold! Five hundred dollars." Haven't had no takers yet. Prolly cause it was dirty. White ball cap, working around airplanes, it's bound to get kinda dirty. So I washed it, which is what this post is all about.
How to keep your Catalina Airport hat nice & clean. And your EAA Life Member Hat too, if you got one. Or that tatty green thing that I wore in Danang or that spiffy red jobbie from Johnston Atoll. (Been there. Did that. But they were outta T-shirts so I got the hat.)
Start with a bucket of water. Bucket has to be big enough to hold your hat, so that means guys with big heads need big buckets. I use a diaper pail. (Because it's there, of course!)
Go down to the grocery store and get yourself some washing soda. Nobody will know what you're talking about if you ask for soda ash or sodium carbonate, even if you tell them you knew Ronny when he was touting for 20 Mule Team, or the fact ‘Trona' is a mineral mined here in California as well as a place to avoid, unless you need some washing soda. Just keep asking. Speak slow. Smile. Tell them it's for a hat.
Yellow box. Arm & Hammer makes it. And if you know about cleaning aluminum, you already know about soda ash so I won't bother telling you its Na2CO3. But I'd better mention it for those other guys.
Cup your palm. No, smaller... about three tablespoonsful per gallon. Now add a dash of regular detergent; mebbe one tablespoonful. Throw it in the bucket. Don't forget the water.
Stir it up good. If you start with warm water washing soda will dissolve pretty quick. Make sure it's all dissolved before you stick in your hat. More than one? Then get a bigger bucket.
Okay, now you can stick in your hat. Poke it down with your hand, this stuff can't hurt you. Unless you're made out of wool. (It's not a wool hat, is it? It is? Then you better pull it out, run it down to the dry cleaners, have them do it.)
Now leave it alone. Oh, you can annoy it now & then, slosh it up & down, do whatever you think might help. But nothing really does. Soaking is what gets it clean; dissolves all that Geezer Pilot Sweat outta the fabric. Sodium carbonate is the secret of success when it comes to greasy geezer sweat.
Warm weather, say seventy or so, I give it about three days. Longer if its cold. Slosh it once or twice.
Got raccoons around your place? Skunks? ‘Possums? Grand kids? Then you gotta cover the bucket, put it somewhere they can't get to it. Otherwise, you'll see your favorite ball cap drug over by the fence, or up in the crotch of a pepper tree. (Life is hard in southern California.)
You got lotsa water? You're going to need at least five gallons to rinse it good. Gallon at a time, with lots of sloshing. No, you don't wring it out; it's a HAT not a diaper. Just slosh it. Take your time (but make sure your hands are clean). Keep sloshing and changing the water until it comes out clear. The water, not the hat.
Dump the water on your flowers. Or onto the compost pile. No phosphates in washing soda. (Which should give you the hint about metal prep.)
To dry your hat you need to block it; to let it sit atop something that will support its shape. I use an old Prince Albert can, painted so it can't rust. Doesn't have to be a perfect match to your head, just something to keep the paneled portion of the hat from collapsing. Now go do something else.
I slosh my hats when I think about it, block them up on a shelf on the shady side of the shop. Takes a couple of days for one to dry.
Clean hat. Now you can go fly. And eat a DC-3.
You know AVX? Prolly not. I've known guys oldern me, spend their whole life buzzing around southern California, never been out to AVX. Which I think is good, especially during the summer when they have the BBQ's up there at Buffalo Bob's (reservations required). It's kinda like those unofficial fly-in's we have now & then, down at Aqua Caliente or the USAAF Training Field, Cibola, California, which ain't on the maps, although AVX is.
DC-3's a hamburger, by the way. No, not the Buffalo Burger, just the plain old fashioned Santa Catalina airport hundred dollar hamburger but with some avocado thrown in. (Ask for extra onion. You'll be glad you did.)
Hint: If you've never been to Catalina, don't go there alone. Find someone who has been there, get them to ride the right seat. If you absolutely, positively gotta go there and never have, land long and stop short, if you can. Otherwise, stay home. Yes, there's a fee; no, there's no fuel or services.
-R.S.Hoover
AV - Poppers & Teenie Two's
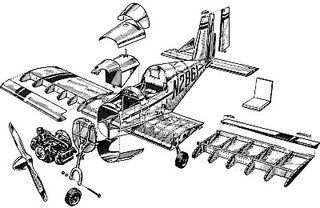
What's a rivet?
Silly question. Everyone knows rivets are those little metal jobbies that hold things together.
Why do you want to hold things together?
Boy! Talk about dumb! Because we don't want them to fall apart, that's why!
Why would they fall apart?
And about there you realize the idiot may know more about stress analysis than you do. Because it's the stresses that make things fall apart and it's rivets that prevent that from happening.
In that ultra-simplistic approach you can think of rivets as stress- or load-paths. When you start thinking of them in that context you will immediately see that the size and type of rivet you use starts to make a lot of sense, along with how many of them you use.
For example, let's say you're up there kicking the bird around and you fall out of a loop a bit too near the ground. To recover you really have to haul on it and end up pulling maybe 3 g's. That means your 650 pound airplane weighed about a ton (!) before you got things straightened out.
The T2's wing has an effective lifting area of about 53 square feet. 2000 pounds divided by 53 is 37.73... nearly forty pounds per square foot.
Out near the tip of your wing the ribs are 18" apart. The spars are about two feet apart so that means some panels of your wing skins have three square feet of surface. Pulling out of that dive, that three square foot panel had to support about 120 pounds.
That's when you begin to wonder, how strong is a rivet? And how many will it take to support 120 pounds?
Would you believe one rivet?
Steel pop-rivets, 1/8" diameter, can safely carry nearly 300 pounds if the load is in shear, meaning the pull is sideways to the rivet.
The truth is, most of the rivets in your wing skins aren't there because you need the strength, they are there to maintain the streamlined shape of the airfoil. If that comes as a surprise, consider the thousands of airplanes that have flown with nothing but a layer of fabric glued to their wooden ribs.
Actually, I'm swinging the lead a bit here because the chord-wise distribution of lift is not uniformly. About half of the load appears in the leading third of the wing and even in fabric-covered aircraft the leading edge is usually something other than fabric.
The point of all this is to quell the doubts many associate with plain old garden variety pop rivets. In the case of the Teenie Two the specified zinc plated steel rivets are more than strong enough.
Just to play the Devil's Advocate, let's take another look at those wing skins and the pop rivets securing them to the ribs and spars. They're spaced about 2" apart out on the ends of the wings. If we leave the spars out of the equation we have a piece of aluminum having an area of three square feet fastened with about 24 rivets. If you assume each rivet can only carry just 100 pounds you will still have enough fastening strength for a load of 2400 pounds. And the 3.5g load was only 120. So you're really pretty safe. In fact, you could use countersunk aluminum pop rivets for your skins and still have a significant safety margin. Save yourself some weight, too. (But only in the skins, please.)
After the load is picked up by the skin it is dumped into to the spars. And after the load is in the spars we have to start calculating the moment of the load as well as its magnitude. If you calculate the load for each inch of the spar's length, you'll see that the caps - the extrusions - are carrying some pretty good stresses. The flush-head aluminum poppers work fine for the skins but the spar has to bear some significant stresses without buckling so you'll want to stick with the 1/8" steel pop rivets. Or something equally strong.
- - - - - - - - - - - - - - - - - - - - - - - - - - - - -
So why did Cal call out 1/8" steel popper for just about everything? Probably because of the convenience. Cal designed the Jeanie's Teenie in the mid-1960's to be built from materials available in any small town. Back then, everyone sold button-head pop rivets and most lumber yards and hardware stores carried various alloys of sheet aluminum in 3'x6' sheets. If you designed a plane to be built using only what's available from your local hardware store then poppers would be the most appropriate choice for fasteners. And zinc or cadmium plated poppers are actually pretty smart in engineering terms because that thin plating of zinc or cadmium is a lot closer to aluminum on the electrolytic scale than is bare steel, stainless steel or Monel. (The closer the elements on the scale, the less electrolytic action between them, thus the less chance of corrosion.)
As further protection against corrosion and as a means of sealing the rivet, Cal said to dip each rivet in anti-corrosion paint from Sears. Unfortunately, he failed to mention the need to wash them first in MEK or lacquer thinner. Such washing is the standard practice when using any rivet that is to receive some form of sealant, since all rivets arrive from the factory with traces of lubricant left over from the manufacturing process. So you rinse it off. So the sealant will stick. (Cal obviously rinsed his rivets before dipping because the original Teenie Two spent most of a quarter-century outside in the weather without any evidence of rusty rivets.)
- - - - - - - - - - - - - - - - - - - - - - - - - - - - - -
If you have a shop filled with riveting equipment and know how to use it, the odds are you'd do exactly that when building a Teenie Two, saving yourself both time and money. And you would probably say as much, from time to time, poking a bit of fun at the newbies who spend more for pop-rivets than you spent on the whole damn airframe :-) (T2 isn't a big bird. Sans engine and landing gear, you're only looking at about a hundred pounds of aluminum.) Or you might point out that using one size and type of fastener (although different lengths) throughout the airframe is not a good example of optimized design. All of which is liable to cause Mr. Newbie to think there is some inherent flaw in using poppers.
There isn't; not when we're talking Teenie Two's. So relax. Properly installed, they work just fine and have for more than forty years in several hundred T2's built in every country except Gondawandaland.
The use of poppers may not reflect optimized design but you should have already guessed that when you saw that Cal used just two thickness of aluminum for everything, notched his ribs instead of fluting them and managed to build the entire airframe without using a single lightening hole. Clearly, this is not an optimized design. But it is an excellent example of appropriate design for an airframe meant to be built by persons having minimum tooling, zero sheetmetal experience and using locally available materials. (Available back then, anyway.)
If you're building a T2 and don't have a lot of aircraft sheetmetal work, stick with poppers, at least for this project. When, as the Mechanic-in-Charge, you elect to use a different fastener, the wiser course is to do so only from a position of knowledge. And, in my opinion, everything you read on the internet (including this) should be considered heresy until it can be personally verified.
Despite the above warning, allow me to offer a bit of experience-based advice: The construction of your Teenie will proceed at a faster pace if you keep in mind the fact Cal's little bird has been flying - safely - for more thirty years. (You can verify that by public records -- his hull number is N2861.) That means there really isn't a lot the rank novice can do in the way of 'improvements.' So quell the urge.
That tenure of service is good evidence that, as designed, the Teenie Two has more than adequate strength. Indeed, in some areas it is far stronger than required. This too may be verified using public records by reviewing the Teenie's accident statistics.
There are some areas of the Teenie's construction that will require your closest attention but on the basis of strength and safety, fasteners is not one of them.
-R.S.Hoover
- - - - - - - - - - - - - - - - - - - - - - - - - - - - - - - - - - - - - - - - - - - - - - - - -
Note: This message was originally posted to the T2 mailing list several years ago. It is being reposted on rah because questions about poppers keep popping up in my mail box.
Subscribe to:
Posts (Atom)